Immuno-oncology, or cancer immunotherapy, investigates how the immune system reacts to cancer, how cancer cells respond, and how the immune system can be manipulated to enhance anti-tumor action. Flow cytometry introduces a powerful and versatile technology into these studies, enabling in-depth analysis of cell signaling and behavior.
Our immune systems are built to protect us from harmful foreign substances, like bacteria and viruses, and abnormal cells, like cancer cells. In the latter case, the job of our immune system is to target and destroy such cells. Sometimes, however, cancer cells find a way to evade the immune response, allowing the cells to grow and spread. This is where immuno-oncology comes in. Research in this growing field focuses on finding treatments that work with our immune systems so that they can function as they should to fight cancer. To learn about the different types of immuno-oncology, read Immunotherapy: The Next Frontier for Cancer and to get a better understanding of current therapies, read Advances in Immunotherapy in Cancer.
Learn about Cancer Immunotherapy Development
Get InfoSince immuno-oncology research focuses heavily on cells, the various types and their behavior in cancer, the use of flow cytometry to interrogate these cells is a no brainer. Flow cytometry allows you to identify cellular markers through surface or intracellular staining techniques. These markers can, in turn, be used to identify different cell types, which can come in handy when developing cell-specific therapies. For example, CD4 is a standard marker for T cell subsets that can also be found on natural killer cells, innate lymphoid cells, and macrophages. It is involved in T cell activation and subsequently provides a foundation for T cell therapies against cancer (Wang et al. 2014).
In addition, flow cytometry easily integrates into immuno-oncology due to its ability to characterize, count, and sort fluorescent-labeled cells based on fluorescence emission. The ability to quantify and characterize specific subsets of cell populations, such as circulating T cells, provides an opportunity to better gauge the immunogenicity of a therapy and enhance our understanding of a complex immune response.
So, what are the steps in immuno-oncology research and how does flow cytometry fit into all of it?
Characterize and Monitor Immune Cells
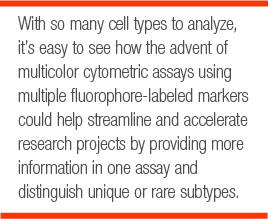
In order to create therapies that can effectively target tumors, researchers need to better understand the various cell types and cellular behavior involved in the immune response to cancer, identify which cells make the best targets for therapies, and characterize the engineered, therapeutic cells to ensure they behave as predicted.
Since immuno-oncology strategies focus on either stimulating immune effector mechanisms or inhibiting suppressive mechanisms, increasing anti-tumor immunity involves the activity of dedicated cell types from all immune response components. These include adaptive immune cells such as cytotoxic T lymphocytes (CTLs) and helper T lymphocytes as well as cells from the innate immune response such as macrophages, dendritic cells (DC), natural killer cells (NK), and granulocytes. Immune suppressive cells including regulatory T-cells (TREGs), myeloid-derived suppressor cells (MDSCs), and tumor-associated macrophages (TAMs) are also involved (Wang et al. 2014). With so many cell types to analyze, it’s easy to see how the advent of multicolor cytometric assays using multiple fluorophore-labeled markers could help streamline and accelerate research projects by providing more information in one assay and distinguish unique or rare subtypes.
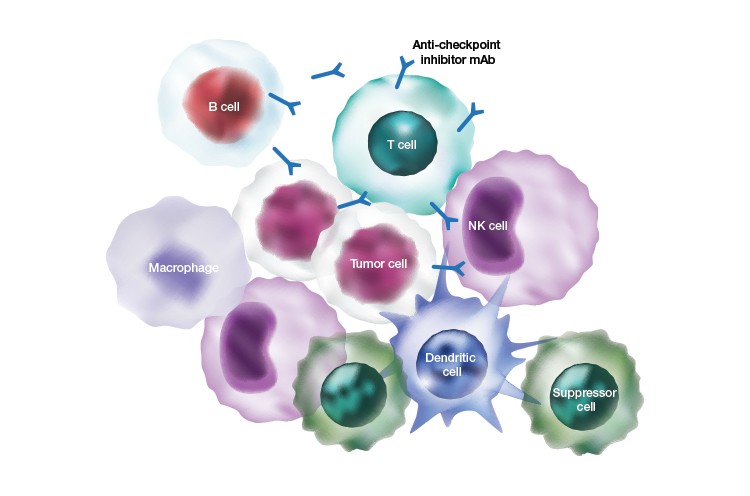
Examples of the various types of cells that can bind to tumor cells.
For example, tumor antigen–specific effector CD4 and CD8 T cells as well as regulatory T cells are highly sought after for immunotherapy studies due to their primary role in the anticancer immune response (Gouttefangeas et al. 2015). Highly sensitive, multiparameter flow cytometers such as the ZE5 Cell Analyzer, when used in combination with validated antibodies conjugated to fluorophores, allow the characterization of these immune cells by enabling the comprehensive evaluation of functional profiles and time-dependent changes in the immune response.
Multiparameter flow cytometry makes detailed analysis of cell subsets possible for all types of immuno-oncology studies. Pairing T cell subset data with functional profiling of the population of interest provides biological relevance to the potential role of a specific cell population. Sorting and functional profiling of T cells by flow cytometry offers highly sensitive, multi-analyte characterization using limited cells. This ensures thorough investigation of precious samples without limitations on what can be observed, extracting a plethora of information from a sample in one assay.
Develop Therapeutic Cells
Engineering T cells or other immune cell subtypes is a primary focus of potential cancer therapies. Gene editing empowers functional changes in cells, turning normal immune cells into cancer fighting machines. Genome editing techniques such as CRISPR can be used to create chimeric antigen receptor (CAR) T cells. These modified T cells are armed with tumor-targeting receptors specific to an individual’s tumor cell antigen and an intracellular signaling domain to drive T cell activation (Ren and Zhao 2017). However, these techniques can be very time consuming since choosing the right transfection method for a cell type that optimizes transfer efficiency and cell viability is significant to the success of the approach.
Flow cytometry systems like the S3e Cell Sorter can enrich for cells of interest and isolate positive edits quickly and efficiently. They can be used to identify rare subsets of successfully edited cells in larger populations, which can then be isolated and studied further. These steps accelerate the CRISPR workflow, reduce costs, and save cell passaging for more viable and robust analysis. See how you can shave 30 days off your gene editing workflow using flow cytometry.
Identify Relevant Biomarkers
While immuno-oncology therapies have increased in popularity due to their use as targeted treatments, not every patient responds well to them. Conventional reliance on pharmacokinetic and pharmacodynamic data cannot offer enough information for consistently successful therapy dosage and scheduling suggestions. Thus, there is heightened interest in identifying predictive and prognostic biomarkers that could improve our understanding of the immune system-cancer relationship to generate more universally reliable treatments.
Flow cytometry is accelerating the process of biomarker detection as an integral part of immuno-oncology therapeutic development, helping to evaluate cancer types and predict clinical responses (Yuan et al. 2016). By identifying biomarkers to further investigate pharmacodynamic changes in cells, an understanding of the potential mechanisms of action and discovery of treatment efficacy and toxicities becomes easier. Advances in flow cytometry have simplified this process by incorporating multiple detectors and lasers into one instrument, reducing the amount of sample needed for a comprehensive biomarker search.
Biomarkers such as programmed cell death protein-1 (PD-1), a checkpoint inhibitor involved in key signaling pathways for cancer cell avoidance and adaptation to host immune surveillance, can be targeted by a drug treatment designed to block its action. The biomarker can then be measured and assessed for efficacy and safety of potential treatments. Research has shown favorable results with antibodies blocking PD-1, expressed on immune cells, or its ligand, PD-L1, which is typically found on tumor cells (Ayers et al. 2017). Using the ZE5 Cell Analyzer provides quantification of these different cell subpopulations with an optimized multicolor panel of known markers. Blocking these suppressive signaling pathways provides long-term and robust anti-tumor behaviors in numerous advanced stage cancers.
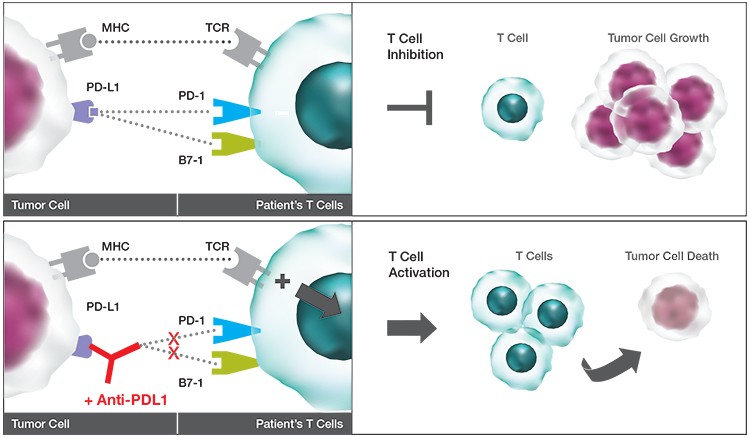
Interaction of PD-L1 with PD-1. The schematic shows how potential therapeutics could promote T cell activation to reduce tumor burden.
Enriching for or isolating a cell type of interest for downstream characterization can easily be performed with flow cytometry. Polychromatic immuno-oncology–specific flow cytometry panels are currently being developed to more efficiently evaluate pharmacodynamic biomarker signatures longitudinally and across therapies. Technologies such as these could become valuable in addressing the inherent complications of assessing response and dosage issues in immunotherapy treatments.
These multiparameter studies examine dynamic changes across heterogeneous immune cell populations and provide highly accurate immunophenotyping of various immune cells (Koch et al. 2008). Large flow cytometry panels ultimately help clarify T cell characterization, revealing the intricate biomarker distribution on single cells and exposing the heterogeneity of a cell population. Biomarker discovery using the Bio-Plex Multiplex Immunoassay System for proteomic studies or the Droplet Digital PCR (ddPCR) System and ddSEQ Single-Cell Isolator for genomic studies further expands the usability of these assays on isolated cell types of interest. Subsequent studies will offer insights into how the immune system as a whole functions in health and disease states and facilitate new biomarker discovery.
Employing specialized flow cytometry technologies and instrumentation such as the ZE5 Cell Analyzer and the S3e Cell Sorter significantly enhances the level and amount of cellular information that can be gleaned from immuno-oncology studies. Furthermore, it enables quicker, easier, and more relevant research. Cumulatively, these advantages can help identify new targets and improve drug design to generate more effective and safer cancer therapeutics.
Download a PDF of this article.
References
Ayers M et al. (2017). IFN-γ–related mRNA profile predicts clinical response to PD-1 blockade. J Clin Invest 127, 2,930–2,940.
Gouttefangeas C et al. (2015). Flow cytometry in cancer immunotherapy: Applications, quality assurance, and future. In Cancer Immunology, N. Rezaei, ed. (Berlin, Germany: Springer International Publishing AG), pp. 471–490.
Koch S et al. (2008). Multiparameter flow cytometric analysis of CD4 and CD8 T cell subsets in young and old people. Immun Ageing 5, 6.
Ren J and Zhao Y (2017). Advancing chimeric antigen receptor T cell therapy with CRISPR/Cas9. Protein Cell 8, 634–643.
Wang M et al. (2014). Current advances in T-cell-based cancer immunotherapy. Immunotherapy 6, 1,265–1,278.
Yuan J et al. (2016). Novel technologies and emerging biomarkers for personalized cancer immunotherapy. J Immunother Cancer 4, 3.
Bio-Rad, Bio-Plex, Droplet Digital PCR, and ddPCR are trademarks of Bio-Rad Laboratories, Inc. in certain jurisdictions.