In December 2022, a groundbreaking law known as the FDA Modernization Act 2.0 (Bill S.5002) was passed by the United States government. This bill grants the U.S. Food and Drug Administration (FDA) the authority to consider data from new alternative methods in the preclinical testing phase for new drug development. Among the new alternative methods to be considered are three-dimensional cell cultures that mimic human tissues and organs, known as microphysiological systems (MPS). Prior to this update, the process for approving a new drug required animal testing followed by human clinical trials to determine whether the drug is safe and effective before market approval. The new law aims to improve clinical outcomes while also decreasing reliance on animal testing.
Passage of this law recognizes two important realities. First, the rapidly maturing field of 3D human cell culture is ready to begin fulfilling its enormous potential for drug discovery applications. Recent advances in microfluidics and stem cell biology have allowed researchers to grow complex biological model systems that reproduce the structures and functions of in vivo organs, making them suitable testing grounds to study the effects of candidate drug molecules.
Second, the limitations of the status-quo preclinical methods, namely 2D cell culture and animal models, are insurmountable and costly. By now, these limitations are well known: 90% of new drug candidates fail clinical trials, largely because the status-quo models either overpredict drug efficacy or fail to fully anticipate toxicity (Sun et al. 2022). These limitations are unsurprising: differences between human and animal physiology are numerous. Drug availability, distribution, and half-life can be affected by many interspecies differences, including breathing and excretion rates, plasma concentration, detoxification pathways, metabolic capacities, and absorption rates in the digestive tract.
Toxicity itself is an organ-specific phenomenon, resulting from a complex sequence of physiological events between cells and drugs, drug metabolites, and drug-protein conjugates. Toxic events cover a spectrum of outcomes, both lethal (apoptosis and necrosis) and sublethal (disruption in cell-cell or cell-matrix adhesion molecules or other injuries that trigger inflammatory response from the immune system). Given this inherent complexity, the need for in vitro model systems that can reproduce and report these adverse effects prior to application in human patients is readily apparent.
What Are Microphysiological Systems?
Three-dimensional cell culture is an umbrella term that refers to the practice of culturing cells in complex 3D environments, in contrast to traditional culturing techniques where cells are grown in two-dimensional monolayers on the surface of culture plates. 3D models are often referred to as microphysiological systems (MPS). These models bring cells into close proximity, where cell-cell interactions heavily influence cell behavior. The development of multicellular model systems has been aided enormously by recent advances in the ability to manipulate pluripotent stem cells in vitro. Pluripotent stem cells can be directed by chemical or physical signals to differentiate into specialized cells in a manner that mimics the in vivo organ formation process.
There are many types of 3D cell cultures, which can be broadly characterized by their different levels of complexity.
Spheroids. Spheroids are generally single cell lines that are cultured without scaffolds in a way that leverages the cells’ natural ability to aggregate and grow in a solid cluster. These are the simplest systems, where cells in the interior of the clusters experience different chemical and physical environments than cells on the exterior. These systems are particularly good models for tumors, as they mimic the solidity that makes drug delivery challenging.
Organoids. Organoids are complex 3D cell cultures where different cell types are cultured in the presence of a scaffold, such as extracellular matrix material, and where chemical signals from cell-cell interactions and cell-matrix interactions direct cell differentiation in a way that creates organ-like structures capable of carrying out organ functions. Because organoids can be established from pluripotent and adult stem cells, these model systems are particularly attractive for use in drug development and personalized medicine, where patient-derived organoids may help predict treatment response and contribute to personalized cancer treatment.
Organ-on-a-chip (OoC) models. OoC systems further increase the ability to culture living-analog systems by growing organoids in the presence of physical forces that mimic human physiology. Typically, OoCs include fluid flow near the culture to simulate vasculature. This fluid flow produces shear and mechanical stress on the tissue and creates chemical gradients around the developing cells. This richly complex environment closely resembles the in vivo environment where tissues develop, and both shear force and chemical gradients can play roles in determining how cells differentiate and organize. By including local cellular, chemical, and physical cues that are all present in the body, organ-on-a-chip systems closely resemble the structure of organs in vivo.
Advanced organ-on-a-chip models. Microfluidic systems can also be designed in ways that chemically connect multiple organoid models, allowing different tissues to interact in ways that reproduce the in vivo environment. From a drug safety perspective, it is of particular interest to grow liver organoids in fluid flow environments upstream of other types of organoids to study how drug metabolites produced by the liver will affect downstream organs. Organoids can also be cultured in the presence of immune cells, either embedded or in the fluid flow, to elucidate inflammatory response in tissues.
Given these model systems’ ability to develop in ways that are analogous to in vivo development, it is apparent why they are so appealing for drug discovery experiments. Efficacy of target molecules can be tested in human cells that are behaving as they would in living organs. Drug molecules are absorbed at rates and doses that better mimic their in vivo behavior, and the long-lasting steady state of 3D models allows for more realistic assessments of the buildup of toxicity over repeated drug exposures. The ability to screen drugs against specific organs — notably liver and heart, where a majority of trials end up failing — will help identify strong and safe candidates earlier in the screening process. 3D cell cultures can reveal toxic events earlier in their course, potentially catching harmful interactions that status-quo models may miss.
Challenges for Using MPS
Despite their great promise, even the most complex microphysiological systems available today are simplified versions of their real-life counterparts, often lacking the full roster of cell types found in actual human tissues and organs. Therefore, there is a significant burden to demonstrate that a model system is producing useful, relevant, and actionable information about organ response to drug treatments. Model systems must be subjected to deep analysis by a suite of tools to assess how well they reproduce the physical and chemical realities of their organ analogs. The suite of tools used for this purpose is familiar to biologists: all of the standard gene expression, protein expression, functional assays, and physical measurement tools are deployed.
Among these tools, multiplex immunoassays are particularly powerful in helping to validate the performance of MPS. First, multiplex assays survey multiple protein targets simultaneously and can provide insight into high-order cellular functions such as protein pathways. These rich datasets can supply meaningful insight into how well MPS are mimicking their in vivo counterparts. Second, these assays are sensitive enough to be run using very little sample, allowing researchers to survey the secreted fluid from an organ model or to biopsy minimal amounts of tissue from these already limited systems. By being minimally destructive, multiplex immunoassays can be deployed at all stages of an MPS drug interaction experiment — helping researchers follow the maturation of the model system and then follow the application of the drug over multiple time points.
The benefits of multiplex immunoassay analysis were demonstrated in a recent study of the ParVivo Human Kidney Chip from Nortis, Inc. (Hoefler et al 2023; Figure 1). In this study, an OoC model of renal proximal tubules was made utilizing human telomerase reverse transcriptase (hTERT)–immortalized kidney cells cultured in the presence of a biologically relevant matrix and continuous perfusion. One group of these model systems was exposed to a known nephrotoxin, polymyxin B, while another was allowed to grow undisturbed. Effluent from both groups was collected at multiple time points and analyzed using a six-target multiplex immunoassay for kidney toxicity (Bio-Plex Pro RBM Human Kidney Toxicity Panel 1). Five of the markers showed upregulation at various time points after treatment with nephrotoxin, while untreated samples showed no change in toxicity markers. Two markers from the multiplex panel were compared to individual ELISA measurements and were found to show the same response profile. Further, data for all six markers were obtained using less total sample (80 µl) than even one ELISA (100 µl). The multiplex assay was able to demonstrate a physiologically relevant response to a known nephrotoxin for this OoC model.
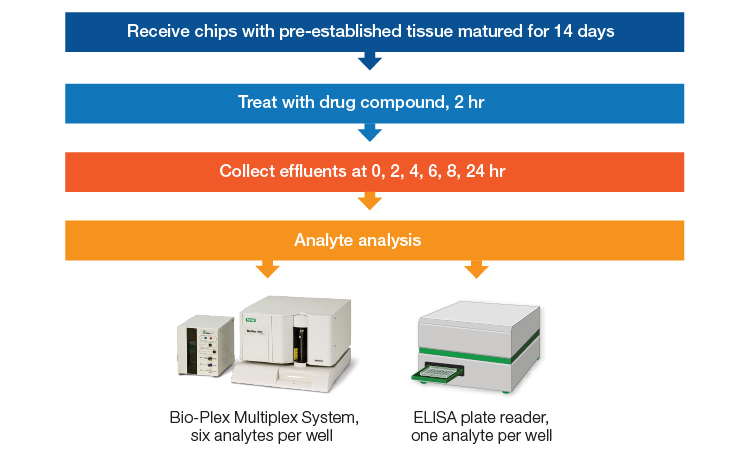
Fig. 1. Design for an experiment comparing an enzyme-linked immunosorbent assay (ELISA) to Bio-Plex Pro Assays for testing of analytes from an organ-on-chip model. Chips with preestablished tissue were matured for 14 days before being treated with the drug compound for 2 hr. Effluent from the chips was collected at 2-hour intervals from 0–8 hours and again at 24 hr after treatment. Analytes were measured using a Bio-Plex Multiplex System, assessing six analytes per well, or using an ELISA, assessing one analyte per well (Hoefler et al. 2023).
Immunocompetent MPS are organoid models that include either embedded or circulating immune cells (or both). Bio-Rad’s multiplex cytokine panels have been used with immunocompetent models to establish the successful inclusion of functional immune cells as well as to verify the validity of the model for studying inflammatory disease. Carroll et al. (2021) developed a murine 3D culture of mixed neural lineage cells that were then seeded with microglia. These immunocompetent models were found to secrete significantly higher levels of cytokines upon exposure to lipopolysaccharide than the unseeded cultures using the Bio-Plex Pro Mouse Cytokine 23-plex Assay.
Gjorevski et al. (2020) engineered an immune-surveilled model of the human gut using a multi-chamber microfluidic system. Three parallel channels allowed the model to sustain an epithelial cell layer, with THP1-derived macrophages embedded in the collagen matrix. By challenging the intact model with bacteria-secreted molecules and monitoring the cytokine release profile with Bio-Plex Pro Human Cytokine and Chemokine Panels, the researchers were able to show that this model behaved like a healthy gut and prevented bacterial translocation. Next, by compromising the barrier integrity using a cytotoxicant, they observed a strong inflammatory response from the embedded immune cells that also stimulated recruitment of circulating neutrophils, which in turn release inflammatory cytokines. This kind of inflammatory crosstalk between multiple cell types has been observed in inflammatory diseases like irritable bowel syndrome (IBS).
Phan et al. (preprint: 2023) developed a lung-on-a-chip model using primary human cell lines, and then compared a healthy version of the model to one created using primary lung cells from patients with chronic obstructed pulmonary disease (COPD). The cytokine expression profile for each model was recorded using the Bio-Plex Pro Human Cytokine 27-plex Assay. Overall, the expression level of all cytokines was higher in the disease model, and importantly, proinflammatory cytokines IP-10 and IL-6 increased significantly. This particular profile has been observed in vivo in response to respiratory viruses, and strongly validates the ability of this lung-on-a-chip model to reproduce in vivo physiology. By profiling the full set of inflammatory cytokines, multiplex panels can provide sophisticated and specific information about how organ models are recapitulating living organs.
The Road Ahead
In order for MPS to fully supplant animal models in preclinical tests, work must continue on many fronts. Biologists must continue to layer ever more complex models and systems together to fully reproduce the functions and behaviors found in living organs. Microfluidic devices that can serve both the microscale world of cells and the macroscale world of tissues and organs need to continue development. Engineering solutions must be found to make microphysiological systems manufacturable at scale.
In addition, reproducibility is a particular challenge in the field, where batch-to-batch variability tends to impact both the cellular components as well as organism-derived matrix materials. Automation and highly rigorous quality control regiments will be required to address these challenges. With their ability to deliver deep biological insight from minimal sample input, multiplex immunoassays, including Bio-Plex Pro RBM Apoptosis Assays, will be crucial tools for validating the performance of these developing model systems and for evaluating the safety and efficacy of drug candidate molecules in preclinical studies.
Check out the full app note for more information about the use of an organ-on-chip with Bio-Plex Assays.
References
Carroll JA et al. (2021). A 3D cell culture approach for studying neuroinflammation. J Neurosci Methods 358, 109201.
Gjorevksi N et al. (2020). Neutrophilic infiltration in organ-on-a-chip model of tissue inflammation. Lab Chip 20, 3365–3374.
Hoefler et al. (2023). Multiplex immunoassay to monitor drug toxicity in renal proximal tubule chips. Bio-Rad Bulletin 3475.
Phan TH et al. (2023). Advanced pathophysiology mimicking lung models for accelerated drug discovery. Research Square. Preprint. https://www.researchsquare.com/article/rs-2473300/v1, accessed March 24, 2023.
Sun D et al. (2022). Why 90% of clinical drug development fails and how to improve it? Acta Pharm Sin B 12, 3,049–3,062.