When testing new drug therapies, it is essential to know the identity of the protein being tested. Whether that protein is the drug itself or the target for the drug, researchers must be absolutely sure of its identity and purity. Though many methods are used in the process of obtaining pure proteins, chromatography is commonly used to separate complex biological mixtures and isolate proteins of interest prior to downstream characterization.
Nowadays, automating chromatography is helping to save time and effort in protein purification. But consulting a chromatogram does not always provide adequate confirmation of protein purity. It is common for two molecules to elute at similar times, resulting in overlapping signals and contaminated sample (Figure 1). Electrophoresis is often a companion technique to chromatography because it allows researchers an orthogonal method with which to visualize the protein species present in a sample. However, adding this method to a characterization workflow can increase the time to results as the elaborate preparation, staining, and imaging steps can be time consuming. Innovations in protein electrophoresis are making this process simpler for lab professionals, with workflows that can be carried out in less than 30 minutes, shaving hours off the original method. One such advancement is stain-free technology. Scientists can characterize drug targets and potential therapeutics with greater confidence when using stain-free gels and gel imaging instruments.
Fig. 1. Purity assessments are an important part of the protein purification workflow. This chromatogram and SDS-PAGE gel highlight the importance of protein purity assessment throughout your purification workflow. The single peak in the chromatogram is resolved further into multiple bands on the SDS-PAGE gel.
The Rise of Therapeutic Proteins
Since the early 1980s, when the first recombinant protein was used for therapeutic purposes, there has been a steady and accelerating rise in the number of protein-based therapeutics. Therapeutic proteins have a broad definition and the term generally encompasses any proteins that can help treat diseases. They are classified according to their potential uses and applications, including those with enzymatic, regulatory, or targeting activity, vaccines, and diagnostics (Leader et al. 2008). They are pervasive throughout the fields of medicine and biotechnology due to their success as effective therapies and their extraordinary potential. Globally, the market for protein therapeutics reached $174.7 billion in 2015, with the U.S. market at nearly $92 billion (BCC Research). Currently, more than 60 FDA-approved protein-based therapies are available, with approximately 140 in clinical trials and more than 500 in preclinical development (Kaspar and Reichert 2013). The overall attractiveness of recombinant proteins as therapeutics means that the ability to effectively formulate and develop them is of the utmost importance.
Recombinant proteins are generally produced in mammalian host cells to ensure that protein therapeutics are correctly folded and have the right posttranslational modifications, such as glycosylation, to ensure they are tolerated by patients. As a result, the therapeutic proteins have to eventually be separated from host cell proteins and other contaminants. One of the most widely used methods for the isolation and purification of recombinant proteins is chromatography.
Protein Purification by Chromatography
Over the past three decades, chromatography, specifically liquid chromatography, has become the technology of choice in the purification of biotherapeutics, due in large part to its resolving power. Various forms of chromatography, including affinity, ion exchange, and mixed mode, are now being utilized to ensure that the final product is as pure as can be without compromising yield. New purification schemes are constantly being introduced and optimized to try and find this ideal balance. Furthermore, the nature of the industry and the fact that these drugs could have significant medical impacts mean that cost and time are also major parameters to consider when looking for the best purification method.
Bio-Rad’s NGC™ Chromatography Systems were designed with this is mind and provide a range of customizable chromatography systems that can increase purification capabilities and be adapted to meet growing throughput needs, obviating the need to buy a new instrument. In addition to the systems’ flexibility and scalability, integrated software provides researchers with powerful system control and the ability to automate multistep purifications and carry out complex separation protocols with minimal hands-on involvement. This ability to easily automate purification methods can save researchers valuable time and effort during complex protein purification workflows.
Despite the versatility and efficacy afforded by chromatography, the technique is not without its drawbacks. One such problem arises from the fact that it is not always possible to determine the purity of a protein just by analyzing a chromatogram. For example, two proteins may elute at similar times, resulting in a single peak on the chromatogram (Figure 1). For this reason, other techniques, such as sodium dodecyl sulfate-polyacrylamide gel electrophoresis (SDS-PAGE), have been combined with chromatography to better elucidate protein purity and yield (Garfin 1990).
Gel Electrophoresis to Determine Protein Purity Prior to Downstream Studies
Sample purity is essential to all protein purification workflows and should be assessed at multiple stages of the process. Verifying sample purity throughout the chromatography purification process lets researchers confidently determine the next steps in their workflow as it helps them identify which fractions to pool, which in turn helps them optimize their chromatography runs. SDS-PAGE analysis is a popular method for verifying the purity of proteins during a protein purification workflow because it can easily separate and detect proteins.
However, SDS-PAGE analysis can be very time consuming. Preparing samples, running the gel, staining and destaining, and imaging can take more than a day. This can add a significant amount of time to a protein purification workflow, especially a multicolumn purification where protein purity is assessed after each column. Due to the highly regulated nature of process-scale protein purifications, it is feasible that standard operating procedures might require purity assessments to be carried out throughout the workflow, which would add a lot of time to the overall process and, in turn, increase the costs associated with protein purification. However, in cases where processes are not so regulated, researchers sometimes prefer to skip the multiple purity verification steps and instead rely on past purifications and other empirical data to pick the appropriate fractions (Raynal et al. 2014), checking the final purity of their proteins only at the end of the purification process. Given the extremely high stakes in drug development and the need for absolute confidence in protein purity, as well as the need to control costs, researchers cannot afford either to spend a lot of time carrying out purifications or to cut corners. To address this particular problem, Bio-Rad has leveraged the use of stain-free technology to significantly reduce the time it takes to check protein purity.
Save Time with Stain-Free Technology
The principle behind stain-free technology is based on the fact that aromatic amino acids like tryptophan can be modified with UV irradiation. Bio-Rad has utilized this technology in its SDS-PAGE gels, where proprietary trihalo compounds incorporated in the gels modify tryptophan residues in the protein sample following UV irradiation. Upon excitation in stain-free enabled imagers, the resulting 58 dalton tryptophan adduct emits a fluorescence signal that promotes the visualization of the protein sample (Yadav et al. 2014). Stain-free gels are no different from regular SDS-PAGE gels and can be used with standard buffers and reagents. However, unlike with Coomassie and other dyes, there are no time-consuming staining or destaining steps as the proteins can be visualized directly.
Studies comparing the standard Coomassie staining method with stain-free gels have shown that procedures utilizing stain-free gels are significantly faster and substantially improve the SDS-PAGE process (Yang et al. 2010). In addition to the significant time savings afforded by the stain-free gels, they offer a lower limit of detection, are more sensitive, and provide a better dynamic range than Coomassie stains (Figure 2) (Hammond et al. 2013, McDonald et al. 2008, McDonald 2009, Taylor et al. 2013). Furthermore, stain-free gels are environmentally safe, as no toxic hazardous organic material is utilized.
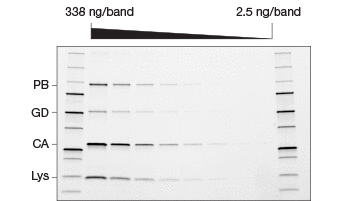
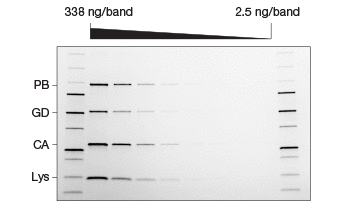
Fig. 2. Comparison of stain-free and Coomassie-stained gels. TGX Stain-Free™ Gels were loaded with standards and a serial dilution of a protein mixture with four different proteins with varying tryptophan (Trp) content: phosphorylase B (PB, 1.4% Trp, 97 kD), glutamic dehydrogenase (GD, 0.8% Trp, 56 kD), carbonic anhydrase (CA, 2.3% Trp, 31 kD), and lysozyme (Lys, 3.4% Trp, 14 kD). Gels were run at 300 V for 20 min and immediately imaged using a stain-free enabled imager (A). Gels were then stained with Coomassie stain and imaged using the same imager (B).
Bio-Rad’s protein purification workflow with the automated NGC Chromatography System and TGX Stain-Free Gels can save researchers a significant amount of time in protein purification, as the stain-free gels provide quick purity and yield checkpoints throughout the chromatography workflow. Specifically, the time needed to run and analyze chromatography fractions can be reduced from more than a day to approximately 25 minutes.
Adoption of Stain-Free Technology
Though the use of stain-free technology in chromatography workflows is relatively new, it is rapidly being adopted by researchers, as it brings significant time savings and efficiency to their protein purification workflows. One such scientist talks about how stain-free technology has helped him overcome some of the challenges in his research.
Dr. Olivier Dalmas, Scientist at NGM Biopharmaceuticals, works on developing technologies with membrane proteins and, as a result, spends a lot of time expressing and purifying them. Membrane proteins are extremely difficult to express and the challenge for him, and where a lot of his effort is concentrated, is in trying to use the best and fastest technology that can give him the results he wants. When carrying out small-scale purifications, he couples all his chromatography steps with SDS-PAGE to find out whether his protein of interest is present and to see how pure it is. “What I like about the stain-free system is the efficiency. It’s way faster than any other staining [method],” Dalmas responded when asked about how stain-free technology is helping his research. Dalmas touted the fact that the gels can be run at very high speeds (300 V) and that he can get his results in as little as 25 minutes, without cumbersome Coomassie staining and destaining. “I think it is a no-brainer for scientists like me to use a system like this, and it really cuts corners where you can, without compromising the quality of the data.”
Conclusions
As the need for protein therapeutics grows, so too will the need for better and more efficient technologies with which to generate, develop, and manufacture them. Chromatography and gel electrophoresis have long been used in workflows from small-scale discovery through to process-scale protein purification workflows; they provide the versatility and integrity required for drug discovery and development. Reflecting the need to shorten development time, automation of purification methods and stain-free technology have been incorporated into protein purification workflows. Stain-free technology has been shown to be a highly effective and superior replacement for standard staining and destaining methods. In addition, both advancements provide a significant time savings for protein purification workflows, making them an efficient and cost-effective addition to the drug development process.
References
BCC Research, “Technology advancements in protein therapeutics spurring market growth,” Press Release (Wellesley, May 25, 2016).
Garfin DE (1990). One-dimensional gel electrophoresis. Methods Enzymol 182, 425–441.
Hammond M et al. (2013). A method for greater reliability in western blot loading controls: stain-free total protein quantitation. bio-rad.com/webroot/web/pdf/lsr/literature/Bulletin_6360.pdf, accessed June 6, 2017.
Kaspar AA and Reichert JM (2013). Future directions for peptide therapeutics development. Drug Discov Today 18, 807–817.
Leader B et al. (2008). Protein therapeutics: a summary and pharmacological classification. Nat Rev Drug Discov 7, 21–39.
McDonald K et al. (2008). In-gel protein quantitation using the Criterion Stain Free™ Gel Imaging System. bio-rad.com/webroot/web/pdf/lsr/literature/Bulletin_5782B.pdf, accessed June 6, 2017.
McDonald K (2009). Overcoming the Coomassie blues. bio-rad.com/webroot/web/pdf/lsr/literature/Bulletin_5939.pdf, accessed June 6, 2017.
Raynal B et al. (2014). Quality assessment and optimization of purified protein samples: why and how? Microb Cell Fact 13, 180.
Taylor SC et al. (2013). A defined methodology for reliable quantification of western blot data. Mol Biotechnol 55, 217–226.
Yadav G and Liu N (2014). Trends in protein separation and analysis – the advance of stain-free technology. bioradiations.com/trends-in-protein-separation-and-analysis-the-advance-of-stain-free-technology, accessed June 6, 2017.
Yang X et al. (2010). Comparison of Criterion™ TGX Stain-Free™ Precast Gel System and standard Coomassie staining procedures for running and imaging protein gels. bio-rad.com/webroot/web/pdf/lsr/literature/Bulletin_6008.pdf, accessed June 6, 2017.